The author(s) will give a talk
Long-term cumulative impacts of a gravel road in an ice-wedge polygon landscape, Prudhoe Bay, Alaska
1 Institute of Arctic Biology, University of Alaska Fairbanks
2 Institute of Arctic Biology, University of Alaska Fairbanks
3 Institute of Northern Engineering, University of Alaska Fairbanks
4 Institute of Northern Engineering, University of Alaska Fairbanks
5 Geophysical Institute, University of Alaska Fairbanks
6 Institute of Northern Engineering, University of Alaska Fairbanks
7 Alaska Ecoscience
8
Introduction and methods: How do the combination of Arctic roads and climate change interact to affect adjacent terrain and vegetation in ice-rich permafrost regions? This study was the first ground-based assessment of the long-term cumulative indirect terrain and vegetation impacts that followed the initial construction of an arctic oilfield road in an ice-wedge-polygon landscape. The direct impact is the “footprint” of the original road. The indirect impacts are those that occur following road construction and include the interactions between climate-related changes and infrastructure-related changes.
This study was preceded by studies that documented extensive regional infrastructure- and climate-related landscape changes in the Prudhoe Bay Oilfield (PBO) (Fig. 1), Alaska (e.g., Raynolds et al., 2014) and was prompted the prospect of more roads, seismic trails and transportation corridors being built to fully develop the oil resources of northern Alaska, including in the National Petroleum Reserve-Alaska (NPR-A) and the Arctic National Wildlife Refuge, AK, which was recently opened to development by the U.S. Congress .
Nearly all roads and most of the permanent infrastructure in northern Alaska are built on thick gravel pads, approximately 1.5 m (5 feet) thick. The thick pads are needed to minimize thermokarst and thermal erosion of the permafrost beneath them. The elevated roads, however, also redistribute snow and water, alter the hydrology of adjacent tundra areas, and add large volumes of road dust to adjacent ecosystems.
The landscapes and vegetation adjacent to roads and other forms of infrastructure are also affected by the impacts of climate change. The discovery of recent abrupt widespread geomorphic changes in ice-wedge-polygon landscapes in Northern Alaska (e.g., Liljedahl et al., 2016) has led to more detailed studies of the processes involved in ice-wedge thermokarst, impacts to nutrient cycling, and trace-gas fluxes.
This study further expands this discussion to the long-term cumulative impacts of a heavily travelled road that crosses an ice-wedge polygon landscape in the PBO. Such roads and landscapes are common in the developed areas of the flat coastal plain of northern Alaska. Previous studies have focused on the historical changes within the oilfield, mainly through the application of remote sensing and integrated geoecological and historical change mapping methods (Raynolds et al. 2014). Many indirect cumulative impacts are difficult to assess solely through the use of remote sensing methods. Recent field data from two sites in the Prudhoe Bay Oilfield (PBO) (e.g., Jorgenson et al. 2015; Kanevskiy et al., 2017; Walker et al. 2015), historical climate data; historical permafrost, landform, soil, and vegetation data (e.g., Walker et al. 1980); an historical aerial photograph record of 42 years between 1949 and 2014 (U.S. Navy: 1949, BP Exploration Alaska 1968–2014), and vegetation-plot data from the 1970s (Walker 1985) were used in the study.
We used ground-based studies and historical data from two sites that are near to each other and to other nearby sites where long-term climate and permafrost data have been collected. The study focused at the Colleen site (CS, Fig. 2) that is adjacent to the most heavily travelled road in the oilfield; the NE (T1) side of road is most seriously impacted by climate change and road dust, and the SW (T2) side is affected by climate change, road dust, and road-related flooding. The Jorgenson site (JS) is relatively unaffected by road-related impacts. We posed two main questions: (1) What were the vegetation and terrain of the of the study sites like prior to oilfield development? (2) How have the cumulative impacts of climate change and the road affected terrain and vegetation at these sites? We examined four scenarios of increasing severity of cumulative impacts (Fig. 3): A. Pre-road (1949–1968); B. Climate change and no road (JS, 1968–present); C. Climate change and road dust (CS, 1968–present); and D. Climate change, road dust, and road-related flooding.
Conclusions and recommendations • Climate-related terrain impacts since the 1970s at the JS and CS were pervasive at both sites and include thicker active layers, large numbers of new thermokarst ponds, increased erosion of the polygon troughs, and greater polygon trough-center microrelief contrasts.
• It is likely that increases in both summer temperatures and precipitation contributed to recent rapid increases in thaw ponds at the JS and CS after approximately 1995.
• Climate-related changes to the vegetation include a likely regional increase in the abundance of dwarf prostrate and erect deciduous willows, and large-scale changes to the distribution of moist, wet, and aquatic vegetation types related to the transformation from low-centered-polygons to transitional and high-centered polygons.
• The large increases in water and aquatic plant communities in polygon troughs require further studies to characterize the plant communities and how they affect the rates of pond succession, trophic structure, food webs, and the degradation and stabilization of ice wedges.
• The road-related impacts included those of heavy road dust, roadside snow drifts, and roadside flooding. The most apparent impact of road dust was the addition of a thick surface soil mineral horizon on top of the original organic surface horizons. This acted to alter polygon morphology near roads. Vegetation impacts from road dust included major reductions in the cover of most plant growth forms and species richness, and the introduction of halophytes near the road probably due to salts used for dust control. The number of species and cover of small forbs, pleurocarpous mosses, and lichens were greatly reduced within 50 m of roads and were apparent well beyond the limits of the 200 m transects in this study.
• Roadside snowdrifts insulate the soils, keeping the tundra relatively warm during winter. The addition of road dust to the snow promotes early melting snow and pond ice, roadside flooding, warmer soils, and deeper thaw near heavily travelled roads. Snow drifts along infrequently travelled roads and pipelines in more remote areas of the oilfield melt much later.
• The terrain impacts of road-related flooding along CS T2 included complex interactions between the flooded polygon troughs and climate-related erosion of the northern shoreline of Lake Colleen that require further study. The result has been wide deeply eroded thermokarst ponds in polygon troughs near the road that are interconnected to the lake.
• The combined climate-related and infrastructure-related impacts were most pronounced along CS T2, where polygon morphologies were most altered, and the vegetation most changed from pre-road conditions. Longer growing seasons, enhanced productivity, and large increases in water bodies have promoted use by waterfowl near roads that have heavy traffic.
It is difficult, possibly impossible, to build large Arctic oilfields on land without extensive networks of pipelines and roads to connect the many drill sites and facilities. The oil industry now has over a half century of experience with building infrastructure in ice-rich permafrost regions. Major advances in technology have reduced the direct footprint of individual development actions, but there continues to be a need to consider the long-term cumulative indirect impacts of infrastructure and climate change in the construction of new roads and pipelines in areas of ice-rich permafrost.
We recommend that future environmental assessments for new large-scale oil developments in areas with extensive ice-rich permafrost include: (a) consideration of the cumulative indirect impacts of climate change and its interactions with land-use change decisions to assess the full likely area of terrain and vegetation impact; (c) conduct multi-year baseline observations and descriptions of the geoecological and geotechnical conditions during the planning phase that include the details of the distribution and structure of ice-rich permafrost, snow, and vegetation; (d) develop landscape sensitivity maps based on this information to identify the most sensitive areas; (e) use a hierarchical perspective to consider the cumulative consequences of climate change and large-scale land-use actions at plot, landscape, regional, and global scales; (f) develop better methods to decrease the effects of road dust, roadside snow drifts, and flooding; (g) consider avoiding construction altogether in particularly valuable landscapes with highly sensitive ice-rich-permafrost.
Jorgenson, M.T., et al., 2015, Role of ground ice dynamics and ecological feedbacks in recent ice wedge degradation and stabilization. Journal of Geophysical Research: Earth Surface, 120(11), pp. 2280–2297.
Kanevskiy, M., et al., 2017, Degradation and stabilization of ice wedges: Implications for assessing risk of thermokarst in northern Alaska. Geomorphology, 297, pp. 20–42.
Liljedahl, et al., 2016, Pan-Arctic ice-wedge degradation in warming permafrost and its influence on tundra hydrology. Nature Geoscience, 9(4), pp. 312–318.
Raynolds, M.K., et al., 2014, Cumulative geoecological effects of 62 years of infrastructure and climate change in ice-rich permafrost landscapes, Prudhoe Bay Oilfield, Alaska. Global Change Biology, 20(4), pp. 1211–1224.
Walker, D.A., 1985, Vegetation and environmental gradients of the Prudhoe Bay Region, Alaska. CRREL Report 85-14, Hanover, NH: U.S. Army Cold Regions Research and Engineering Laboratory, p. 240.
Walker, D.A., et al., 2015, Infrastructure-Thermokarst-Soil-Vegetation Interactions at Lake Colleen Site A, Prudhoe Bay, Alaska. [online] AGC Data Report, Fairbanks, pp.1–100.
Walker, D.A., et al., 1980, Geobotanical atlas of the Prudhoe Bay region, Alaska. Hanover, NH: Cold Regions Research and Engineering Laboratory, CRREL Report 80-14.
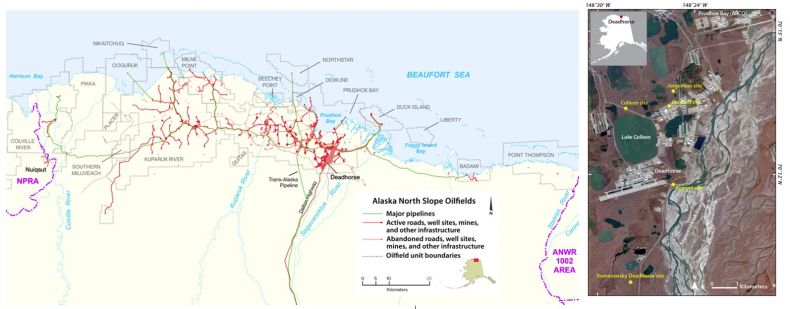
Fig 1.
Left: The North Slope oilfields and major infrastructure, 2010. Red lines: gravel roads, airstrips, and construction pads. Green lines: major pipelines. Note the Iñupiat village of Nuiqsut in the National Petroleum Reserve-Alaska (NPRA). Development has expanded into NPRA since 2010. The Trans-Alaska Pipeline System (TAPS) and the James Dalton Highway link the oilfields to Fairbanks, AK. Base map courtesy of BP Exploration-Alaska. Right: Deadhorse, AK region in the eastern portion of the Prudhoe Bay Oilfield. The infrastructure includes two airports, numerous drill sites and support facilities that were constructed on elevated gravel pads designed to protect the permafrost from thawing. Permafrost research has been conducted at the Colleen site, Airport site, Jorgenson site, Gas Arctic site, and the Romanovsky Deadhorse site. The Colleen site is located along a straight section of the Spine Road, 2.9 km north of the Deadhorse airport. Climate data were collected from the Prudhoe (ARCO) station (1969–1985) and Deadhorse station (1986–2019). The image is a false-color infrared image derived from multispectral satellite data. Google Earth, 9/6/2014, ?Maxar Technologies 2020.
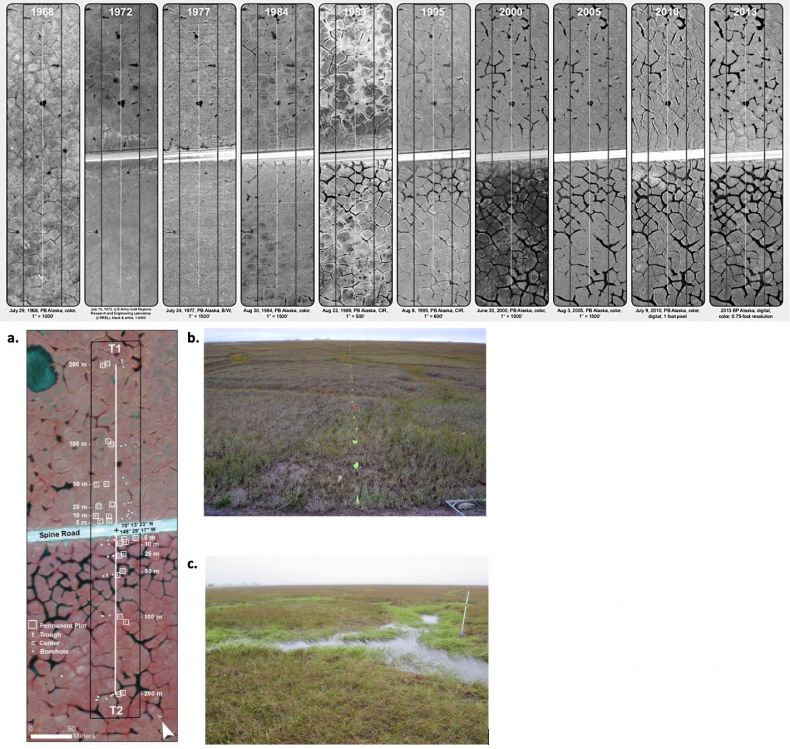
Fig 2.
Top: Time series (1968–2013) of changes to ice-wedge polygons at the Colleen Site. The images are a subset of the full record of 42 images between 1949-2014. The 1968 photo was taken before construction of the Spine Road, which is the white band through the center of the 1972–2013 photos. Vertical white lines are the 200-m transects. The black rectangles denote the primary area of analysis along the transects. Aerial photographs: courtesy of the BP Alaska Exploration. Bottom: Transects, plots, and permafrost boreholes at the Colleen site (CS). a. Digital false-color infrared aerial photograph (9 Aug 2010) of the 200-m transects (white lines): T1 (NE side of the road) and T2 (SW side). Paired vegetation plots (open white squares) are located in polygon troughs (t) and centers (c) at approximately 5, 10, 25, 50, 100, and 200 m from the road. Permafrost boreholes (open white circles) are located in troughs and centers at similar distances. b. Ground view in 2014 of Transect T1 on the relatively well-drained side of the road. Pin flags at 1-m intervals are for periodic monitoring of vegetation and environmental factors. Heavy road dust is apparent on this side of the road. c. Transect T2 on the flooded side of the road, where the troughs between polygons are eroded and filled with water. The white 1.5-m stake has iButton® thermistors at 0-, 10-, 25-, 50-, and 100-cm heights. Similar stakes are located at 0 m, 25 m, 50 m, 100 m, and 200 m on both sides of the road.
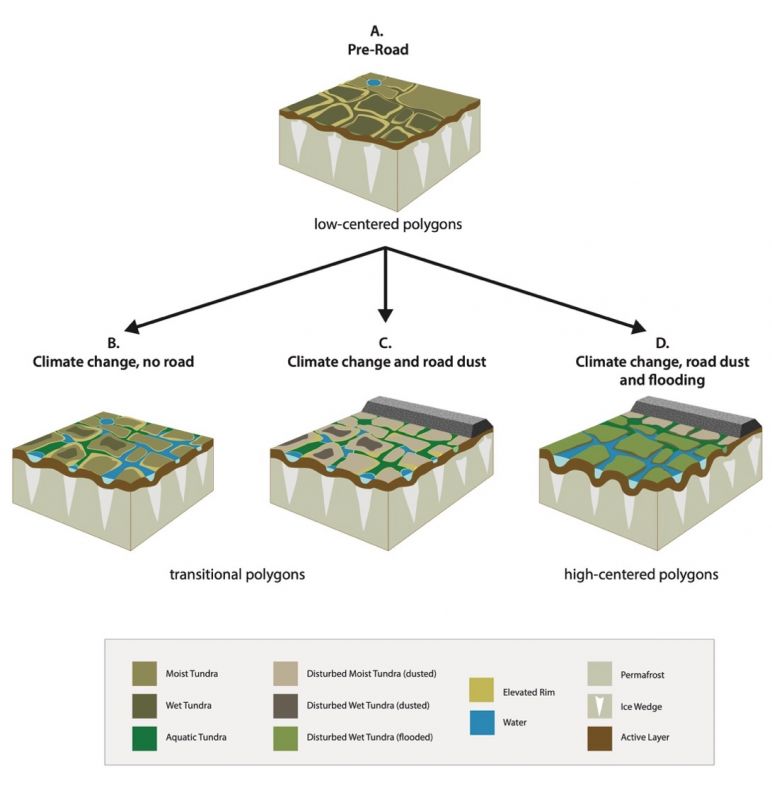
Fig 3.
Four scenarios of terrain and vegetation transitions due to climate change and a gravel road. A. Pre-road (1949–1968); interpreted mainly from aerial photographs, geobotanical maps and ground observations by the IBP Tundra Biome in the 1970s; B. Climate change, no road (1969–present), interpreted mainly from information at the JS; C. Climate change and road dust (1969–present), interpreted mainly from CS T1; and D. Climate change, road dust, and flooding (1969–present), interpreted mainly from CS T2.